Motor function after stroke is promoted by early neural network reorganization

Authors: Mihaela Molnar, Oana Vanta
Keywords: neurorehabilitation, repetitive transcranial magnetic stimulation, neuronal plasticity
Introduction
Is motor function after stroke is promoted by early neural network reorganization? Stroke is a common cerebrovascular disease with high morbidity and mortality worldwide, representing the leading global contributor to adult disability. However, after severe neurological impairments, most patients show some degree of sensory and motor function restoration. It has been suggested that brain plasticity or the structural and functional modifications of neuronal constituents and networks is responsible for this spontaneous functional recovery [1].
To learn more about stroke research, visit:
- Accelerating the process of rehabilitation through virtual reality: The standing point of Reh@Task in post-stroke disability
- The feasibility of repetitive sensory stimulation in the rehabilitation of the upper limb after a stroke (the PULSE-I Study)
Or watch some interviews with top-notch experts:
- Prof. Natan Bornstein (Chair of Israeli Stroke Society)
- Prof. Marc Fisher (President of the World Stroke Organization)
Remodeling neuronal structures and networks at various levels, from single cells to large-scale networks, facilitates recovery from motor cortex lesions after stroke. Within hours following ischemia, there have been noticed changes in growth factors (critical molecules for tissue repair and regeneration), transmitter systems (e.g., glutamate and gamma-aminobutyric acid transmitter systems), and axonal sprouting (early growth of axon with an important role in neuroplasticity, neuronal development, and recovery of injured neurons). Neuroimaging studies at the system level showed that the connectivity of the primary motor cortex (M1) with remote areas is decreased in the first few weeks post-stroke but then rises along with motor recovery [2]. Neuronal reorganization after a stroke is observed in functional imaging studies, which provide information about changes in the stimulus or task-induced activation patterns in the affected (ipsilesional) and opposite (contralesional) hemispheres of the brain [1]. The finding that modifications in network connectivity are associated with neurological dysfunction has inspired the concept of “correcting” the dysfunctional network architecture utilizing noninvasive brain stimulation techniques, such as repetitive transcranial magnetic stimulation (rTMS) [2].
According to a proposal made by the Stroke Roundtable Consortium [3], the time after a stroke was divided into phases:
- Hyperacute phase – first 24 hours
- Acute phase – first 7 days
- Early sub-acute phase – first 3 months
- Late subacute phase – 4-6 months
- Chronic phase – from 6 months onwards [3] (Figure 1).
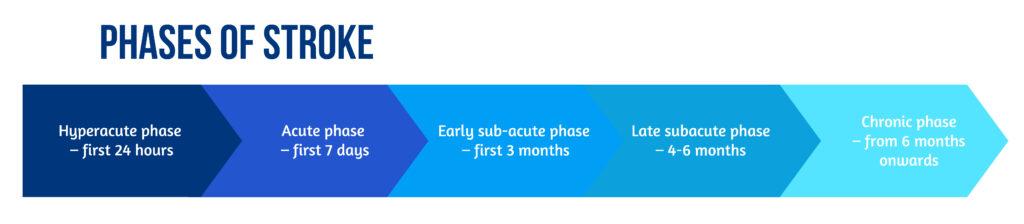
This distinction is made because post-stroke mechanisms associated with recovery are time-dependent. The most dramatic improvements happen in the first few weeks following a stroke, frequently hitting a relative plateau after three months marked by less-significant recovery rates, especially concerning motor symptoms [3]. A cascade of plasticity-enhancing processes causes the effects presented in Figure 2 [3].
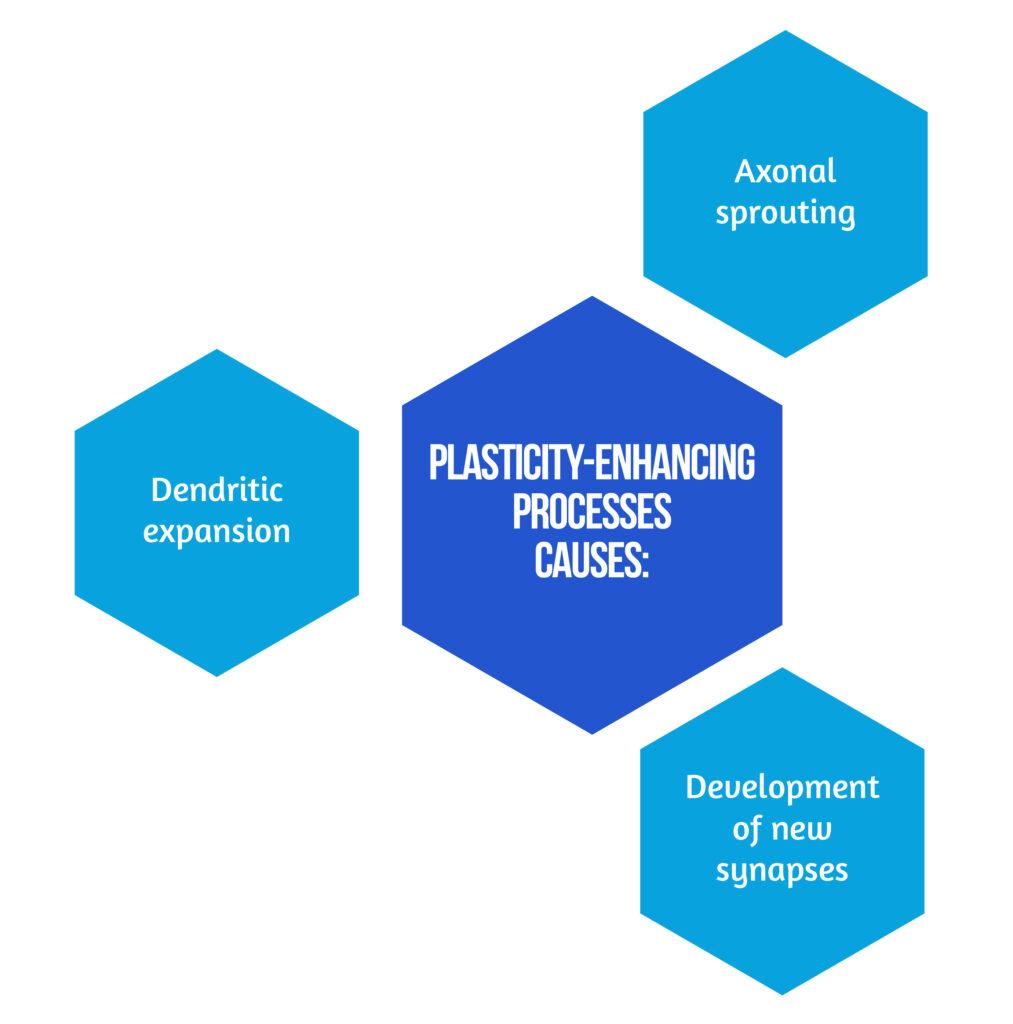
Neurological abnormalities following a focal stroke lesion are influenced by the widespread failure of interconnected brain systems and the local dysfunction at the injury site. It is possible to reconcile earlier localized neuroimaging findings with known anatomical connections of sensory function to a considerable extent because of the evidence for functional and effective connectivity behind clinical symptoms [4].
Implications of Repetitive Transcranial Magnetic Stimulation (rTMS) in Neurorehabilitation
TMS (transcranial magnetic stimulation) is a particularly relevant method to evaluate the central nervous system (CNS) because it non-invasively activates superficial cortical cells via a transient time-varying magnetic field [5]. Therefore, repetitive TMS may help modify local plasticity and enhance the lesioned motor system’s connectivity, which is commonly diminished after stroke [2].
In animal models, rTMS can stimulate synaptic plasticity through different processes, as showcased in Figure 3.
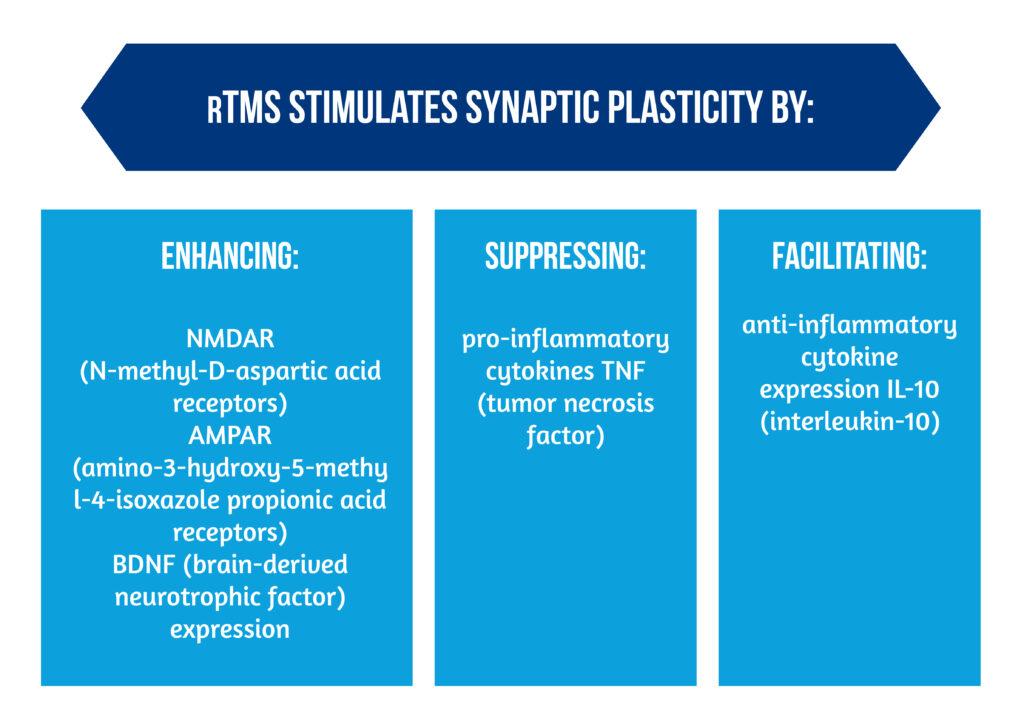
Additionally, the release of angiogenesis-related factors TGF (transforming growth factor) and VEGF (vascular endothelial growth factor) can contribute to vasculogenesis and angiogenesis (see Image 1) [6].
Recently, rTMS has become popular as a novel form of neurological rehabilitation therapy, and prior studies have shown that it is effective in reducing motor impairment following stroke [7].
The aim of the study “Shaping Early Reorganization of Neural Networks Promotes Motor Function after Stroke”, conducted by L. J. Volz et al., was to demonstrate that motor recovery of the paretic hand can be enhanced by repeated applications of iTBS (intermittent theta-burst stimulation) over ipsilesional M1, therefore ameliorating stroke-induced neuronal network dysconnectivity [2]. They used maximum grip strength as their main outcome parameter to assess motor recovery. The grip force is a key component of regaining hand motor function; it can be measured quickly and easily while maintaining high standards and is resistant to compensatory movement techniques because the primary movement direction cannot be significantly changed by enlisting other muscles [2].
The authors conducted a sham-controlled, pseudo-randomized, single-blinded study that enrolled twenty-six patients with first-ever ischemic stroke resulting in unilateral hand motor deficit [2].
Pseudo-randomization made sure that both groups were balanced for the following factors known to influence motor recovery and outcome:
- Age
- Initial severity of the hand motor deficit
- The number of days after stroke onset [2].
Four patients received M1 stimulation, and six received control stimulation after the initial 10 patients enrolled in the trial were randomly allocated to a treatment group. Accordingly, all subsequent patients were assigned to the alternative treatment group after being matched to the patient who met most of the randomization criteria (1-3). In order to eliminate any selection bias, patient matching was carried out by an experimenter who was not involved in the patient’s behavioral, electrophysiological, or neuroimaging assessments. Those were used one day before the initial stimulation (baseline) and one day after the last stimulation to measure hand motor performance, cortical excitability, and resting-state fMRI (post-intervention) [2]. The eligibility criteria are showcased in Figure 4.

The primary outcome parameter was the maximum grip strength knowing that grip performance is a key component of regaining hand motor function, it can be measured quickly and easily, and it is resistant to compensatory movement techniques. Because of this, improvements in grip strength represent a sensitive behavioral marker reflecting the restoration of neurological function rather than compensatory learning of new movement patterns, and it has been directly related to M1 activity [2].
The grip strength and cortical excitability were evaluated by relative grip strength and the Jebsen-Taylor Hand Function Test (JTT) because both involve device-dependent quantification [2]. Jebsen-Taylor Hand Function Test (JTT) uses simulated activities of daily living to provide a standard and objective assessment of fine and gross motor hand function (ADL). It has been demonstrated that determining grip strength using a flexible ball vigorimeter as opposed to a static dynamometer is less reliant on hand anthropometry [2].
Cortical excitability was evaluated by measuring the amplitudes of motor-evoked potentials (MEPs) induced by neuronavigation single-pulse TMS. The muscle activity was measured using surface electrodes placed on the paretic hand’s abductor pollicis brevis muscle [2].

The intervention in both groups consisted of the same experimental protocol applied to all the subjects. It included iTBS directly preceding physiotherapy on 5 consecutive days, with a slight difference between the groups in the stimulation location: the M1-stimulation group received ipsilesional M1-stimulation, and the control-simulation group received parieto-occipital vertex stimulation, see Figure 5 [2].
The coil was tilted at a 45° angle and touched the skull not with its center but with the rim at the opposite side of the handle in order to minimize any potential effects of cortical stimulation in the control condition. The electromagnetic field is significantly weaker and outside the goal range in this position, but the typical TMS skin sensation is preserved because the coil-cortex distance is virtually greater. A recent study that used this method found no distinction between actual and sham stimulation in terms of how it was perceived. Because it was used as a between-subject design of TMS-naïve patients, it was not known the difference between M1 and control-stimulation groups [2].
Compared to control stimulation during subacute stroke, priming physiotherapy with iTBS given to ipsilesional M1 dramatically improved the recovery of grip strength [2].
Functional Magnetic Resonance Imaging was used to evaluate resting-state blood oxygenation level-dependent activity, which consists of a monitorization by an infrared camera attached to the end of the scanner. The instructions for the patients were to remain motionless and to fixate on a red cross on a black screen. For a functional localizer, task patient performed a motor task that required blocks of unimanual actions that were visually cued rhythmic fist closures. In the next block of trials, written instructions for 2 s indicated whether the left or right hand was to be moved. Fist closures were required from subjects for 15 seconds at a blinking circle’s frequency, followed by 15 seconds of resting [2].
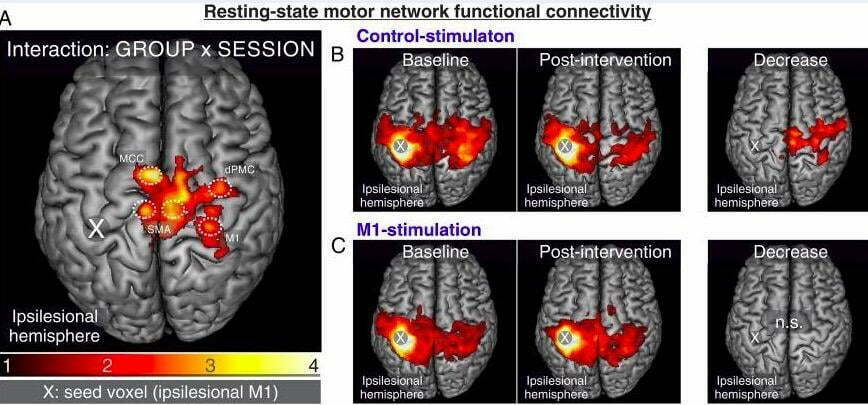
Functional connectivity (FC) of the resting-state motor network was significant between ipsilesional M1 and bilateral cortical motor network, as seen in Figure 6A. Patients with successful motor outcomes showed an increased connection between M1 and each of these areas. Baseline connectivity maps indicated no significant differences between control-stimulation (image 6B) and M1-stimulation groups (image 6C). A clear reduction in the functional connection between ipsilesional M1 and contralateral motor cortex can be seen in the control-stimulation group post-intervention, but no appreciable reduction in the M1-stimulation group. The M1-stimulation group (image 6C) showed connection increases at an uncorrected level rather than a substantial drop, as seen in Figure 6 [2].
Recently research has demonstrated that the contralesional postcentral gyrus is a primary somatosensory cortex center that receives information from the spinal cord and medullary motor neurons [7]. The research of Chen et al. examined functional connectivity (FC) recovery in stroke patients with a completely or partially paralyzed hand, showcasing the following:
- Decreased FC in the contralesional central gyrus and ipsilesional motor areas, indicating that FC retention in the contralesional postcentral gyrus is associated with better recovery.
- The activation of the contralesional precentral gyrus (PreCG.CL) and contralesional postcentral gyrus (PoCG.CL) brain areas in stroke patients’ brain areas are connected with the restoration of motor function.
These findings imply that the alterations in FC between intra- and inter-hemispheric motor networks were responsible for the positive effects of paired inhibitory-facilitatory rTMS on motor dysfunction. Furthermore, for early-stage cerebral stroke patients receiving coupled rTMS, FC alterations were substantially linked with the restoration of motor function [7].
The Perspective of rTMS as an Adjuvant Therapy for Motor Impairment After Stroke
The study of Volz et al. highlights that rTMS affects not only the neuronal characteristics of the stimulated region but also the activity levels of distant yet related brain regions. Similarly, it has been shown that a single application of iTBS to M1 in healthy patients enhances the site’s resting-state connection with distant motor regions (ipsi- and contralateral premotor cortex) [2].
At the network level in patients who suffered a first-ever stroke, M1 stimulation appears to have stopped the decline in motor system connectivity measured at rest that was seen in individuals who received control stimulation. Inferring that stimulation-induced increases in connectivity may reflect a brain mechanism supporting the recovery of grip strength in subacute stroke, less reduction in functional connectivity was linked with better outcomes. It was found that iTBS enhance neuroplasticity and motor network connectivity, therefore ameliorating diaschisis and promoting early recovery of motor function [2].
Following an early-stage ischemic stroke, transcranial magnetic stimulation treatment is a potential approach for adjuvant rehabilitation therapy [7].
References
- van Meer MPA, Otte WM, van der Marel K, Nijboer CH et al. Extent of Bilateral Neuronal Network Reorganization and Functional Recovery in Relation to Stroke Severity. Journal of Neuroscience 2012;32 (13) 4495-4507; doi: 10.1523/JNEUROSCI.3662-11.2012
- Volz LJ, Rehme AK, Michely Nettekoven JC et al. Shaping Early Reorganization of Neural Networks Promotes Motor Function after Stroke. Cerebral Cortex 2016;26: 2882–2894; doi: 10.1093/cercor/bhw034
- Grefkes Christian, Fink R. Gereon. Recovery from stroke: current concepts and future perspectives. Neurological Research Practice 2020. doi: 10.1186/s42466-020-00060-6
- Westlake KP, Nagarajan SS. Functional conectivity in relation to motor performance and recovery after stroke. Frontiers in Systems Neuroscience 2011. doi: 10.3389/fnsys.2011.00008
- Beaulieu LD, Milot M-H. Changes in transcranial magnetic stimulation outcome measures in response to upper-limb physical training in stroke: A systematic review of randomized controlled trials. Annals of Physical and Rehabilitation Medicine 2018; 224-234; doi: 10.1016/j.rehab.2017.04.003
- Xing Y, Zhang Y, Li C, Luo L et al. Repetitive Transcranial Magnetic Stimulation of the Brain After Ischemic Stroke: Mechanisms from Animal Models. Cell Mol Neurobiol 2022. doi: 10.1007/s10571-022-01264-x
- Chen Q, Wenjun W, Sun H, Zhang H et al. The effect of coupled inhibitory-facilitatory repetitive transcranial magnetic stimulation on shaping early reorganization of the motor network after stroke. Brain Research 2022. doi: 10.1016/j.brainres.2022.147959