Locomotion training after spinal cord injury- the physiological basis of neurorehabilitation

Authors: Ioana Stanescu, Irina Benedek, Oana Vanta
Keywords: neurorehabilitation, spinal cord injury, neuroplasticity, locomotion training
- Spinal cord injuries
- The neuronal basis behind locomotion
- The role of neuroplasticity in locomotor training
- Evidence of a locomotion center in the human spinal cord
- Importance of the physiological basis behind the locomotor ability
- Patients with complete SCI have benefits after locomotion training
- Sensory stimulation in spinal cord injuries
- Conclusions
- References
Spinal cord injuries
A spinal cord injury (SCI) is a significant medical issue and represents a severe cause of morbidity and disability. The axons of the nerves that pass through the spinal cord are damaged; therefore, impairment of motor, sensory and autonomic functions below the lesion is present. Approximately 250 000 to 500 000 new cases are reported annually worldwide, and patients aged 16 to 30 years old are the most exposed to this risk [1].
The most common causes of spinal cord injury are described in Figure 1 below:
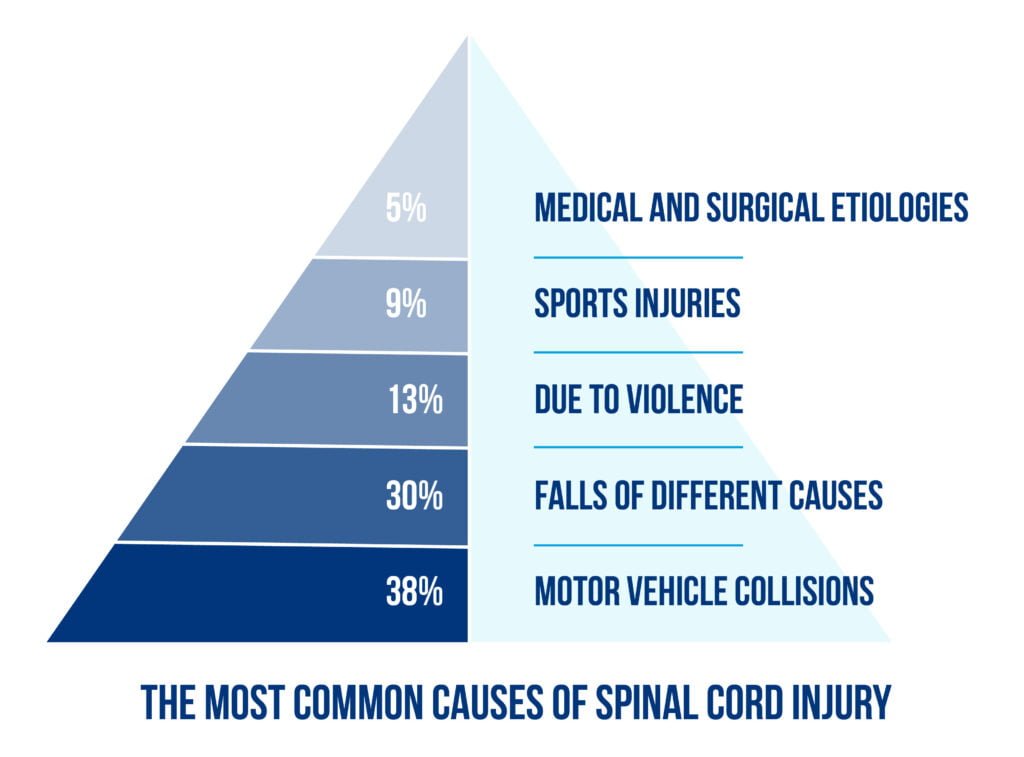
The main goal of neurorehabilitation of spinal cord injuries is regaining independence and achieving a good quality of life. According to patients, this relates to restoring the upper limbs’ motor integrity and the bladder and bowl’s normal function [2]. Nonetheless, recovery of the locomotor ability is one of the main goals. It is highly recognized that the central nervous system is able to regain locomotor function after an incomplete SCI through specific training on treadmills with partial body weight support [3].
A literature review covering the physiological basis of locomotor training after SCI was conducted by Michèle Hubli and Volker Dietz [4], focusing on the studies of neuroplastic changes at the spinal level. The authors assessed 89 articles covering a period from the 1980s to 2010s, covering both human and animal trials, to analyze locomotor training after spinal cord injury to create a novel translatory neurorehabilitative approach [4].
The neuronal basis behind locomotion
The review highlights the importance of locomotor training after spinal cord injury and discusses the relevance of multi-pronged neurorehabilitative approaches and the optimization of using task-specific sensory cues to generate the locomotor pattern [4]. After thorough research, studies demonstrated that the fundamental neuronal circuits responsible for initiating efficient walking patterns are located in the lumbosacral spinal cord. They have played an important role in stepping ability in both animal models and patients who suffered an SCI and are known as “central pattern generators” (CPGs) [5].
Even though CPGs are the primary key in walking initiation patterns, they are insufficient. Feedback from other neurological structures such as visual, vestibular, and proprioceptive systems is crucial. Moreover, supraspinal control is essential to provide coordination in a challenging environment [6]. Recent advances in neuroimaging techniques demonstrated that different cortical areas, such as the medial primary sensory-motor cortices and the supplementary motor areas, are activated during locomotion. The size of the activated regions depends on the person’s walking speed. Furthermore, challenging tasks like walking over obstacles required additional cortical control [7].
The role of neuroplasticity in locomotor training
Neuroplasticity represents the ability of the nervous system to change and adapt after an injury. The effects of Neuroplasticity are described in Figure 2 below [8].
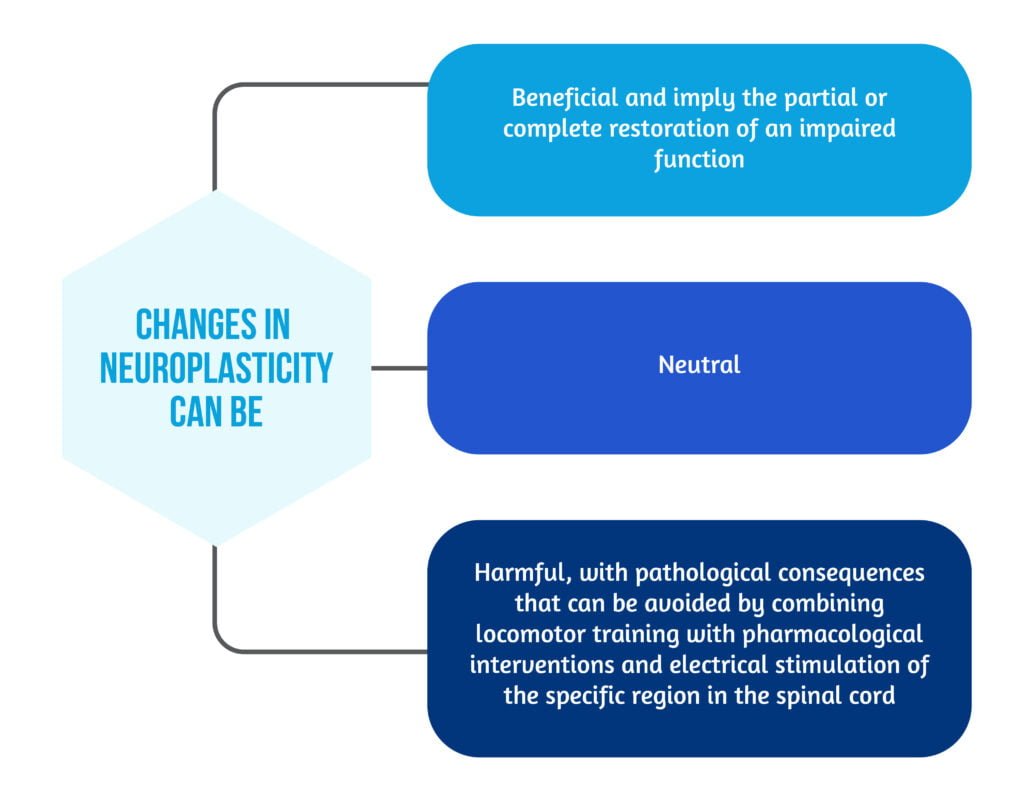
Two essential mechanisms support neural plasticity:
- Regeneration or collateral sprouting, which implies the idea of neurogenesis and synaptic plasticity
- Functional reorganization [8].
The main objective of modern recovery is not just to compensate for disability in patients with SCI but rather to functionally recover their locomotor capacity by using neural plasticity and repair. The spontaneous reorganization of cortical levels was observed up to a year after suffering from a spinal cord injury [4]. Besides spontaneous neuroplasticity, active locomotor training could also generate reorganization at cortical levels. It is essential to mention that structures such as the cerebellum or brainstem also play a key role in rehabilitation and neural plasticity along with spinal neuronal circuits, which are located below the level of injury and can be activated. Therefore, despite focusing on improving different clinical impairments, rehabilitation should exploit the plasticity of neuronal circuits.
Furthermore, the authors [4] pinpointed the animal experiments to support spinal cord plasticity, as experimental models on cats with SCI found that they could regain complete weight-bearing locomotion on a treadmill after 2-3 weeks of daily training. Their analysis [4] also found arguments for the existence of spinal circuitries that control rhythmic and coordinated leg movements in a stepping pattern, as animal experiments proved that the isolated loci in the spinal cord could generate those patterns [4, 5].
Evidence of a locomotion center in the human spinal cord
Available data present some factors indicating the presence of locomotion centers at the spinal cord level. Newborn babies have step-like movements in lower limbs (spontaneous or triggered). This activity in newborns is more likely produced at the spinal cord level because corticospinal projections are not fully grown and myelinated at this age [9]. Moreover, in patients with complete spinal cord trans-section, rhythmic leg movements appeared in sleep or after stimulation [10]. Also, the spinal cord can generate a locomotor pattern using intrinsic circuitries for stepping patterns and sensory feedback information. The complex function of the spinal indicates that polysynaptic spinal reflex pathways integrate afferent sensory inputs with the spinal locomotor pattern [4].
Importance of the physiological basis behind the locomotor ability
Understanding the physiological basis is essential in regaining locomotor ability because the principles of motor learning are the base of spinal rehabilitation programs through task-oriented approaches:
- Task-specific interventions
- Task-variability training
- Continuous feedback
SCI patients’ main achievement after locomotor training was a coordinated pattern of muscle activation in both lower limbs following partial body weight support during driven gait on a moving treadmill [4]. During assisted locomotion, EMG detected muscle activity in incomplete SCI patients is modulated in a stepping pattern, similar to muscle activation during normal walking [4].
Patients with complete SCI have benefits after locomotion training
Patients with complete paralysis below the lesional level must be permanently assisted during training by physiotherapists or robotic devices. Full bodyweight support is mandatory during robotic-assisted walking; those patients are prone to falls. The benefits of assisted locomotor training are described in Figure 3 below [11]:
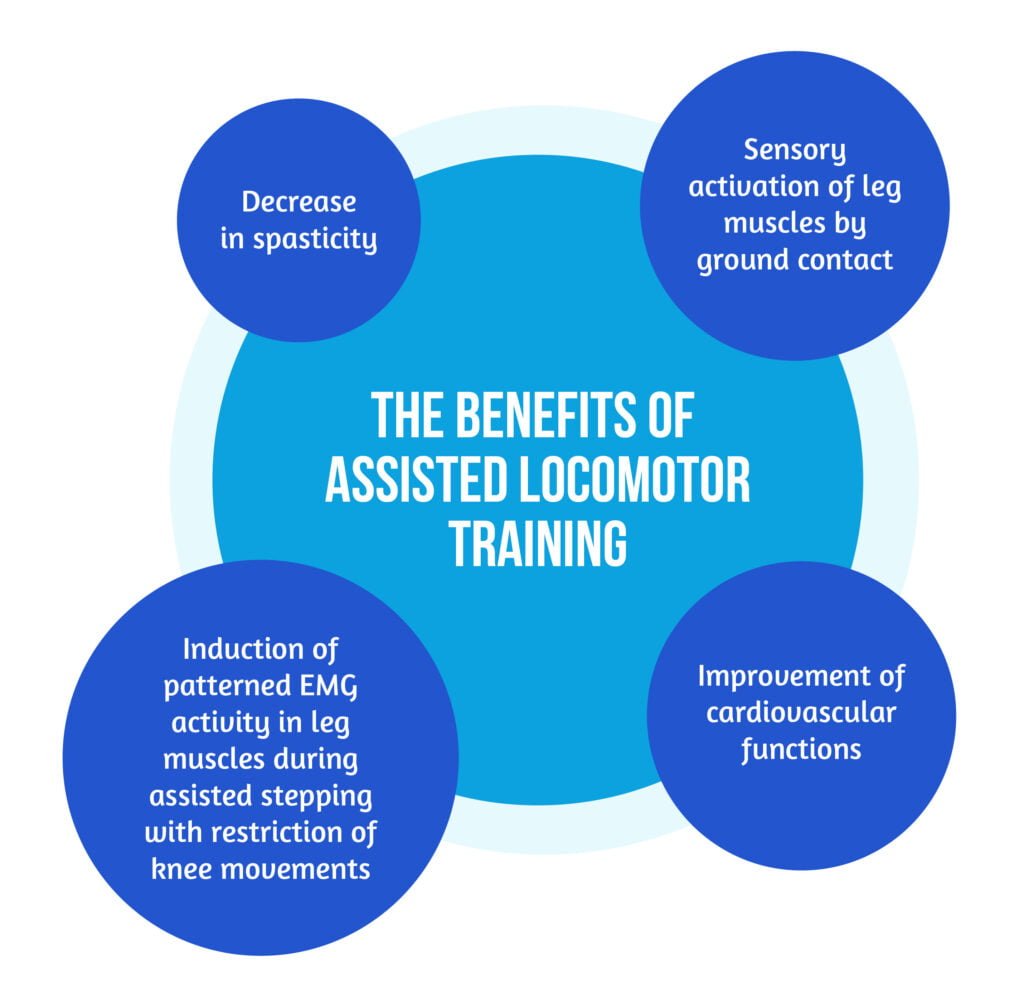
In general, for better results, locomotor training should always be challenging for patients but there is a need for only minimal support provided either by therapists or robotic devices [8].
The locomotor training program efficacy relies on the following factors:
- It is beneficial for complete and incomplete SCI
- The timing for starting the program is still under debate (early-onset could be beneficial)
- Program intensity needs to be established by further studies
- A better effect could be obtained with a longer duration (dose-response relationship) [4].
Sensory stimulation in spinal cord injuries
After a spinal cord injury, voluntary and involuntary motor pathways and sensory ones can be partly spared and play a key role in the recovery of locomotion. Nevertheless, partially preserved corticospinal and/or propriospinal pathways remain the basis for generating a locomotor pattern due to the intrinsic capacity of spinal locomotor circuitries and the sensory feedback from receptors located in joints, muscles, tendons, and skin [4].
It is considered that sensory activation is essential in lower limbs motor training because:
- Spared proprioceptive pathways in incomplete SCI are relevant in the gait rehabilitation process;
- Spinal cord intrinsic pattern generators are activated by sensory cues from specific receptors during normal walking;
- Reduced sensory feedback impairs and delays locomotor recovery [12];
- Artificial activation of sensory inputs will facilitate the generation of a locomotor pattern.
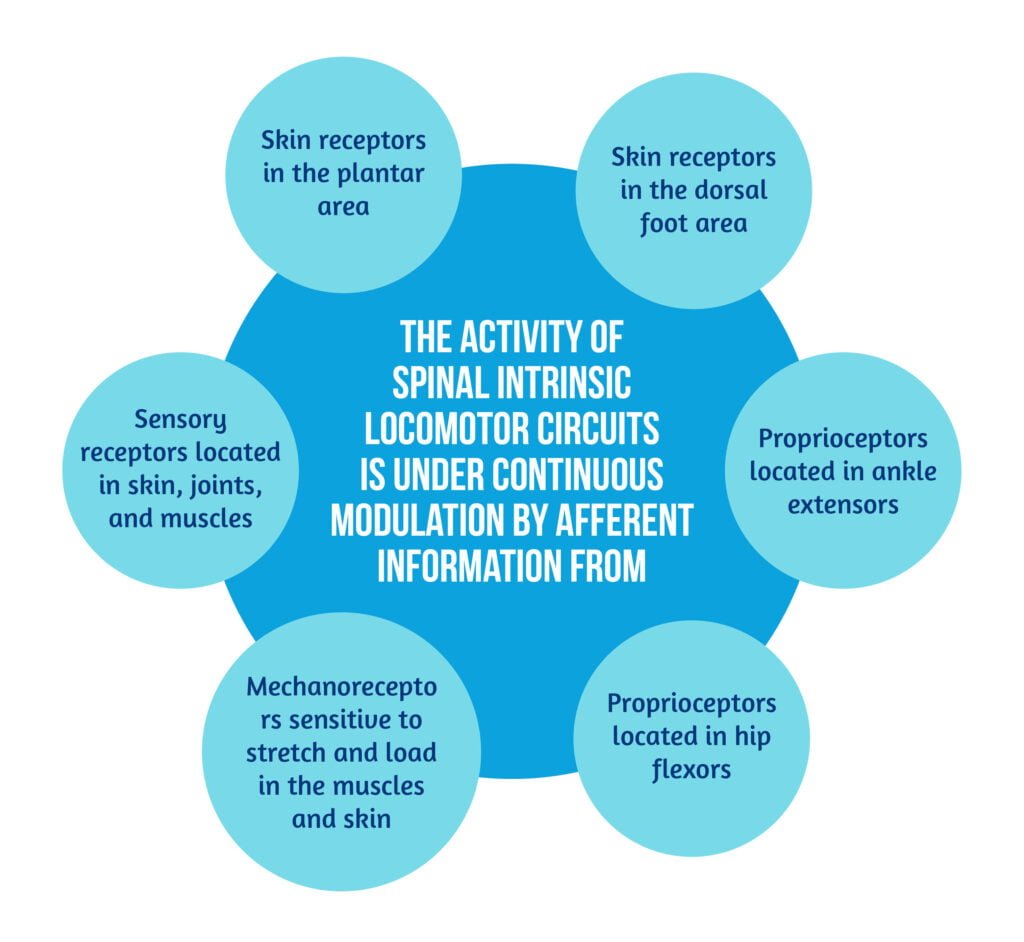
Continuous sensory input in the spinal cord is mandatory for the stimulation of spinal circuitries. Thus, strategies for sensory activation that impact spinal locomotor training were studied [4]. Rehabilitation strategies used to stimulate the spinal cord via sensory pathways are described in Figure 5 below [13–23]:

The sensory information, either through direct stimulation of sensory receptors in the skin of the feet while sustained or through direct electrical stimulation of the peripheral nerves or spinal cord, is thought to be integrated into polysynaptic spinal reflex pathways that modulate the autonomous locomotor pattern. After a spinal cord injury, this integrated circuit is at the base of locomotor rehabilitation programs.
Conclusions
Considering the extensive review of Michèle Hubli and Volker Dietz [4], one can conclude that the know-how on complex neurorehabilitation strategies after a spinal cord injury relies on understanding the physiological basis of locomotory mechanisms and the implications of sensory feedback and is essential in implementing sustainable SCI rehabilitation programs.
The authors [4] also highlight the importance of complex neurorehabilitation strategies that efficiently improve SCI patients’ locomotor function. In the rehabilitation process of SCI patients, it is essential to use task-specific locomotor training as a part of an individual center-based approach as well as task-specific sensory cues. Spinal sensory activation facilitates the generation of a stepping-like pattern and is a key feature of training locomotor recovery. Spinal sensory stimulation can be achieved by external electrical stimuli applied at the level of the spinal cord or peripheral nerves.
Thus, sensory stimulation should be used in new neurorehabilitative approaches to facilitate locomotor pattern generation after a spinal cord injury and warrants further investigations in clinical trials.
References
1. Bennett J, M Das J, Emmady PD. Spinal Cord Injuries. [Updated 2021 Aug 26]. In: StatPearls [Internet]. Treasure Island (FL): StatPearls Publishing; 2022 Jan-. Available from: https://www.ncbi.nlm.nih.gov/books/NBK560721/
2. Anderson KD. Targeting recovery: priorities of the spinal cord-injured population. J Neurotrauma 2004, 21(10):1371–1383 DOI: 10.1089/neu.2004.21.1371
3. Barbeau H, McCrea DA, O’Donovan MJ, Rossignol S et al. Tapping into spinal circuits to restore motor function. Brain Res Rev 1999, 30(1):27–51. DOI: 10.1016/s0165-0173(99)00008-9
4. Hubli, M., Dietz, V. The physiological basis of neurorehabilitation – locomotor training after spinal cord injury. J NeuroEngineering Rehabil 10, 5 (2013). https://doi.org/10.1186/1743-0003-10-5
5. Grillner S. Neurobiological bases of rhythmic motor acts in vertebrates. Science 1985, 228(4696):143–149. DOI: 10.1126/science.3975635
6. Rossignol S, Dubuc R, Gossard JP. Dynamic sensorimotor interactions in locomotion. Physiol Rev 2006, 86(1):89–154. DOI: 10.1152/physrev.00028.2005
7. Suzuki M, Miyai I, Ono T, Oda I et al. Prefrontal and premotor cortices are involved in adapting walking and running speed on the treadmill: an optical imaging study. Neuroimage 2004, 23(3):1020–1026. DOI: 10.1016/j.neuroimage.2004.07.002
8. Puderbaugh M, Emmady PD. Neuroplasticity. [Updated 2022 Feb 8]. In: StatPearls [Internet]. Treasure Island (FL): StatPearls Publishing; 2022 Jan-. Available from: https://www.ncbi.nlm.nih.gov/books/NBK557811/
9. Forssberg H. Ontogeny of human locomotor control. I. Infant stepping, supported locomotion and transition to independent locomotion. Exp Brain Res 1985, 57(3):480–493 DOI: 10.1007/BF00237835
10. Lee MS, Choi YC, Lee SH, Lee SB. Sleep-related periodic leg movements associated with spinal cord lesions. Mov Disord 1996, 11(6):719–722. DOI: 10.1002/mds.870110619
11. Dietz V, Muller R, Colombo G: Locomotor activity in spinal man: significance of afferent input from joint and load receptors. Brain 2002, 125(12):2626–2634. DOI: 10.1093/brain/awf273
12. Harkema SJ. Plasticity of interneuronal networks of the functionally isolated human spinal cord. Brain Res Rev 2008, 57(1):255–264. DOI: 10.1016/j.brainresrev.2007.07.012
13. Gurfinkel VS, Levik YS, Kazennikov OV, Selionov VA. Locomotor-like movements evoked by leg muscle vibration in humans. Eur J Neurosci 1998, 10(5):1608–1612. DOI: 10.1046/j.1460-9568.1998.00179.x
14. Selionov VA, Ivanenko YP, Solopova IA, Gurfinkel VS. Tonic central and sensory stimuli facilitate involuntary air-stepping in humans. J Neurophysiol 2009, 101(6):2847–2858 DOI: 10.1152/jn.90895.2008
15. Gerasimenko Y, Gorodnichev R, Machueva E, Pivovarova E et al. Novel and direct access to the human locomotor spinal circuitry. J Neurosci 2010, 30(10):3700–3708. DOI: 10.1523/JNEUROSCI.4751-09.2010
16. Minassian K, Persy I, Rattay F, Pinter MM et al. Human lumbar cord circuitries can be activated by extrinsic tonic input to generate locomotor-like activity. Hum Mov Sci 2007, 26(2):275–295 DOI: 10.1016/j.humov.2007.01.005
17. Harkema S, Gerasimenko Y, Hodes J, Burdick J et al. Effect of epidural stimulation of the lumbosacral spinal cord on voluntary movement, standing, and assisted stepping after motor complete paraplegia: a case study. Lancet 2011, 377(9781):1938–1947. DOI: 10.1016/S0140-6736(11)60547-3
18. Lapointe NP, Guertin PA. Synergistic effects of D1/5 and 5-HT1A/7 receptor agonists on locomotor movement induction in complete spinal cord-transected mice. J Neurophysiol 2008, 100(1):160–168. DOI: 10.1152/jn.90339.2008
19. Domingo A, Al-Yahya AA, Asiri YA, Eng JJ, Lam T. A systematic review on the effects of pharmacological agents on walking function in people with spinal cord injury. J Neurotrauma 2012, 29(5):865–879. DOI: 10.1089/neu.2011.2052
20. Cogiamanian F, Vergari M, Pulecchi F, Marceglia S, Priori A. Effect of spinal transcutaneous direct current stimulation on somatosensory evoked potentials in humans. Clin Neurophysiol 2008, 119(11):2636–2640. DOI: 10.1016/j.clinph.2008.07.249
21. Winkler T, Hering P, Straube A. Spinal DC stimulation in humans modulates post-activation depression of the H-reflex depending on current polarity. Clin Neurophysiol 2010, 121(6):957–961. DOI: 10.1016/j.clinph.2010.01.014
22. Cogiamanian F, Vergari M, Schiaffi E, Marceglia S et al. Transcutaneous spinal cord direct current stimulation inhibits the lower limb nociceptive flexion reflex in human beings. Pain 2010, 152(2):370–375. DOI: 10.1016/j.pain.2010.10.041
23. Cortes M, Thickbroom GW, Valls-Sole J, Pascual-Leone A, Edwards DJ. Spinal associative stimulation: a non-invasive stimulation paradigm to modulate spinal excitability. Clin Neurophysiol 2011, 122(11):2254–2259 DOI: 10.1016/j.clinph.2011.02.038